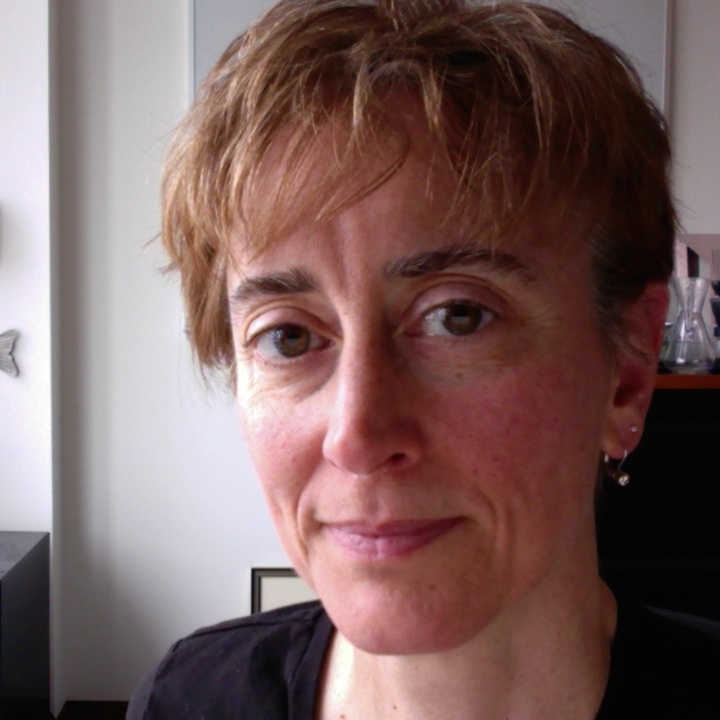
Shannon Fisher, M.D., Ph.D.
Associate Professor of Pharmacology, Physiology & Biophysics
Director, Laboratory of Developmental Therapeutics
Research Interests:
Craniofacial defects, affecting formation of the face and skull, affect 1/1000 children and are one of the most common categories of birth defects. Although sometimes these disorders are caused by single gene mutations, in most cases the risks are more complex, likely due to multiple genes and environmental factors, and the mechanisms poorly understood.
Dr. Fisher uses the zebrafish as a model organism to study disorders of the skeletal system, with a particular focus on craniofacial development. We have developed genetic tools and imaging methods to study the skull, and are applying these to better understand the mechanisms of human craniofacial development and the genetic risks underlying craniofacial birth defects.
Current Research:
Tools and methods to image skull development
We have developed methods to carry out serial confocal imaging of individual fish, to capture the dynamic events of skull formation. Using this approach, we have created publicly available datasets of bone, cartilage, and osteoclast distribution during skull development.
Genetic risk for craniofacial disorders
My group pioneered the use of zebrafish to analyze transcriptional and regulatory elements from the human genome, and have identified and studied dozens of enhancers associated with skeletal genes. Using these and other powerful tools for genome editing, we are exploring the genetic risk for craniofacial disorders in humans, identified through genome–wide association studies or sequencing of patient cohorts. In particular, we are working to understand the mechanism of genetic risk associated with several genes in the BMP signaling pathway.
Genetic screen for new craniofacial mutants
A primary advantage of zebrafish is the ability the perform large–scale genetic screens for mutations affecting development. Over a period of several years, my group carried out a screen for mutations disrupting skull formation, and identified >20 novel phenotypes. We have mapped several of these to regions of the genome, and are working to identify the specific mutated genes.
Research accomplishments:
Zebrafish skeletal development and mutant analysis
During my postdoctoral studies, I made the unexpected discovery that mutants in the BMP inhibitor chordin were viable to adulthood, and showed severe patterning defects in the vertebral column. I demonstrated that the pool of somitic precursor cells was expanded as a result of unregulated BMP signaling, and that the expansion, and the resulting adult skeletal defects, were corrected by restoring chordin function in the early embryo. Early genetic screens in zebrafish had identified many mutations affecting craniofacial cartilages during the first several days of development, but my description of chordin mutants was the first of a skeletal phenotype beyond the larval period, and specifically in bone. I pioneered the use of radiography to characterize adult skeletal defects in the chordin mutants, and subsequently used it to identify another late–acting skeletal mutation in chihuahua, one of the genes encoding type I collagen. Significantly, the chihuahua mutant is an accurate genetic and phenotypic model for human osteogenesis imperfecta, and helped to firmly establish zebrafish as a model for human skeletal diseases.
Transcriptional regulation in skeletal development and disease
My lab was the first to localize osteoblast precursor populations in the zebrafish embryo, based on expression of the runx2 genes. The question of how these precursors are induced has motivated a major area in my research program, to exploit comparative genomics and the zebrafish to understand the genetic regulation of osteoblast differentiation. While at Hopkins, I collaborated with my colleague Andrew McCallion to use zebrafish to test conserved sequences from the human genome for enhancer activity. Mouse transgenesis is expensive and labor–intensive, severely limiting the number of sequences that can be analyzed, but cell culture assays lack critical spatial and temporal information. Our approach offers a practical compromise, allowing us to screen many more sequences than feasible in the mouse while preserving spatial and temporal information. The idea that extremely conserved non–coding sequences, highly similar from mammals to fish, were likely to be functional enhancers was widely accepted. Our conceptual breakthrough was to show that sequences only conserved among mammals could nonetheless function as appropriate tissue–specific enhancers in zebrafish, paving the way to use zebrafish as an efficient system to identify and study mammalian enhancers.
Focusing on genes critical in skeletal development and disease, we have tested >300 sequences and identified >30 active enhancers from 12 genes. One striking finding is the frequency with which multiple enhancers with similar or overlapping activities are associated with a single gene. The most extreme example is the 11 independent enhancers associated with the gene encoding Aggrecan, the most abundant non–collagenous protein in cartilage. We also find little correlation with location; most tissue–specific enhancers are not in proximal upstream regions and are often quite distant from their associated genes. This collection of enhancers represents a unique resource. The transgenic lines are invaluable for visualizing skeletal cells in live animals, and have led to genetic tools for long–term lineage studies of the zebrafish skeleton. We also developed a vector system based on ΦC31 recombinase that permits efficient replacement of coding sequences in established transgenic lines, and will allow us to measure quantitative changes in gene expression resulting from enhancer sequence variants. The identified enhancer sequences have provided insight into regulation of individual genes. In particular, we identified three RUNX2 enhancers, which all direct expression to bone, but are not similar in sequence and respond to different regulating signals and pathways. We found that separate RUNX2 enhancers are regulated by FGF and Wnt signaling, directly linking these pathways to bone formation in vivo, and allowing us in future studies to identify specific signals responsible for inducing bone formation in the embryo. These same pathways are likely to act in maintaining bone in the adult, and therefore also provide a direct link between RUNX2 regulation and degenerative skeletal diseases.
Ongoing work and future goals
Identification of new genes required in skeletal development Human birth defects frequently affect the craniofacial skeleton, and for most of these the genetic and developmental causes are unknown. In our most recent work, we have been laying the foundation for zebrafish as a model to explore the basis of craniofacial defects, in particular those affecting the bones of the skull vault and the sutures joining them. Even in cases of genes known from mouse or human mutations, investigations in zebrafish offer new insights, as shown by our characterization of skull and suture defects in osterix/sp7 mutants, a gene identified as required for mouse bone development over 10 years ago. Although sp7 is expressed in all bones, and mineralization defects are widespread, the zebrafish mutants display surprisingly specific skull defects, including irregular sutures and numerous ectopic skull bones. At the sutures, normal sites of new bone formation during skull growth, the early osteoblast precursor cells proliferate excessively and fail to down–regulate runx2. Our data suggests that their behavior is regulated by BMP signals from adjacent differentiated bone, disrupted in the absence of sp7. This is a new model for the regulation of bone formation at the sutures, and has important implications for our understanding of developmental disorders affecting the skull.
A primary advantage of the zebrafish system is the ability to perform genetic screens, revealing new components of genetic pathways. We have two ongoing efforts to identify genes contributing to skeletal development. Together with my colleague Mary Mullins, we are screening a collection of defined mutants2 that lack embryonic phenotypes, for defects as adults, including skeletal abnormalities visible by X-ray. Over 5 years we will screen mutants for ~3000 genes, or about 12% of the zebrafish genome. Eventually we aim to expand this screen to the remaining collection of mutants, complementing the description of embryonic phenotypes with information about later gene function. In my own lab, we have undertaken a forward genetic screen to identify mutations affecting skull formation and other aspects of the juvenile skeleton. In two years of screening, we have identified >25 mutants with phenotypes ranging from specific isolated skull malformations to total lack of mineralized bone. These new mutants will form the foundation for many years of fertile research into mechanisms of skeletal development.
Imaging and manipulating skull development
The zebrafish is continuously accessible throughout development and nearly transparent at larval stages, and therefore supremely suited for imaging dynamic processes. However, as pigment cells develop and the skin matures in juvenile fish, imaging in either live or fixed specimens is a much greater challenge. The vault of the skull forms during the juvenile period, and offers further imaging challenges because of its complexity and the intricate interrelationships among a large number of skeletal elements. These are best viewed and conceptualized through 3-dimensional reconstructions. Building on our transgenic resources to visualize cartilage and bone through fluorescent labeling, we have developed methods of tissue preparation and microscopy to allow such 3-D reconstructions during skull formation. I was recently funded as part of the FaceBase Consortium through NICHD, together with Dr. Matthew Harris at Harvard University, to generate an online atlas of normal and abnormal zebrafish skull formation. An additional component of the grant is to develop genetic tools to manipulate cell populations during skull development. Building on our successful track record, my lab will generate tissue–specific transgenic lines for genetic lineage studies and for mis–expressing coding sequences, fostering detailed analysis of gene function in skull formation.
Zebrafish and complex human disease
Several genome wide association studies (GWASs) have revealed genetic contributions to complex diseases affecting the skeleton. The vast majority of GWASs fail to identify a biological mechanism, because most genetic variation lies outside coding sequence. The region encompassing one of our identified RUNX2 enhancers has been associated with height variation, bone mineral density, and osteoarthritis risk. In collaboration with Eleftheria Zeggini’s group, we are examining human sequence variations in the region to look for differences in enhancer activity or function that contribute to osteoarthritis risk. More recently, we have established a collaboration to explore genetic contributions to craniosynostosis. Dr. Simeon Boyadiev’s group recently reported a GWAS for sagittal craniosynostosis, in which they uncovered two links, one near BMP2 and one within the BBS9 locus. However, BBS9 is adjacent to the gene encoding BMPER, a known modulator of BMP signaling. We hypothesize that the disease risk is in fact due to variation in a distant regulatory element of the BMPER gene. As the first step towards uncovering the functional basis of the genetic link, we are using zebrafish to identify the regulatory element. Our experimental paradigm offers a powerful approach to this difficult problem, using zebrafish to efficiently identify and characterize human enhancers in regions associated with disease risk. In the future, we aim to extend our studies to other regions of the genome associated with skeletal diseases.
Lab Page
Publications
Recent News