Glossary for Culture Transformation
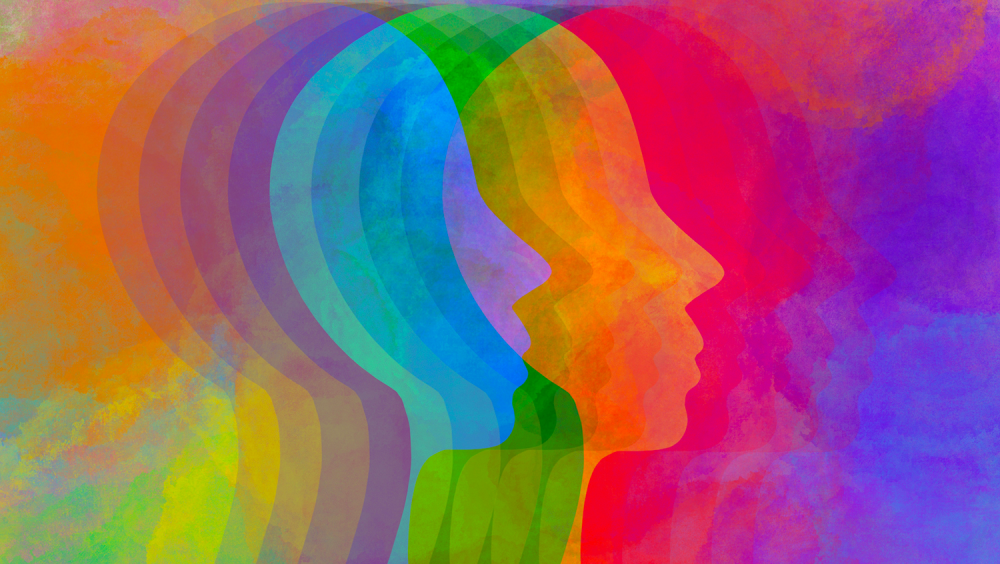
Language reflects our values, shapes our thoughts and actions, and creates common understanding. Launched Nov. 15, the Glossary for Culture Transformation strives to promote a culture of justice, equity and belonging on the Medical Campus. To learn more, read this BMC HealthCity article.